Palladium-catalyzed C–C cross-coupling reactions (Suzuki–Miyaura, Negishi,Stille, Sonogashira, etc.) are among the most useful reactions in modern organic synthesis because of their wide scope and selectivity under mild conditions. The many steps involved and the availability of competing pathways with similar energy barriers cause the mechanism to be quite complicated. In addition, the short-lived intermediates are difficult to detect, making it challenging to fully characterize the mechanism of these reactions using purely experimental techniques. Therefore, computational chemistry has proven crucial for elucidating the mechanism and shaping our current understanding of these processes. This mechanistic elucidation provides an opportunity to further expand these reactions to new substrates and to refine the selectivity of these reactions.
During the past decade, we have applied computational chemistry, mostly using density functional theory (DFT), to the study of the mechanism of C–C cross-coupling reactions. This Account summarizes the results of our work, as well as significant contributions from others. Apart from a few studies on the general features of the catalytic cycles that have highlighted the existence of manifold competing pathways, most studies have focused on a specific reaction step, leading to the analysis of the oxidative addition, transmetalation, and reductive elimination steps of these processes. In oxidative addition, computational studies have clarified the connection between coordination number and selectivity. For transmetalation, computation has increased the understanding of different issues for the various named reactions: the role of the base in the Suzuki–Miyaura cross-coupling, the factors distinguishing the cyclic and open mechanisms in the Stille reaction, the identity of the active intermediates in the Negishi cross-coupling, and the different mechanistic alternatives in the Sonogashira reaction. We have also studied the closely related direct arylation process and highlighted the role of an external base as proton abstractor. Finally, we have also rationalized the effect of ligand substitution on the reductive elimination process.
Computational chemistry has improved our understanding of palladium-catalyzed cross-coupling processes, allowing us to identify the mechanistic complexity of these reactions and, in a few selected cases, to fully clarify their mechanisms. Modern computational tools can deal with systems of the size and complexity involved in cross-coupling and have a continuing role in solving specific problems in this field.
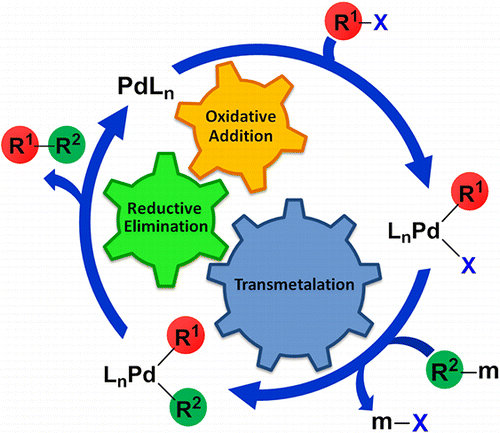